Current Projects
Photopolymerization of Lyotropic Liquid Crystalline Systems
Lyotropic Liquid Crystals (LLCs) are self-assembled surfactant-solvent systems capable of forming a range of mesophases (micelles, micellar cubic, bicontinuous cubic, hexagonal, lamellar) depending on the concentration of the amphiphillic species (Figure 1A). This self-assembly can be used as a template to obtain nanostructured polymers (Figure 1B). However, the use of this technique to create structured polymers is limited as a result of poor nanostructure retention following polymerization. In our work, we investigate photopolymerization as a means of preparing LLC templated polymeric materials. In comparison to other methods of polymerization, photopolymerization are temperature independent and typically occur at rapid rates. Based on these advantages, we have examined the use of photopolymerization in the preparation of LLC templated materials for applications in medicine, separations, and electrochemistry (Figure 1C).
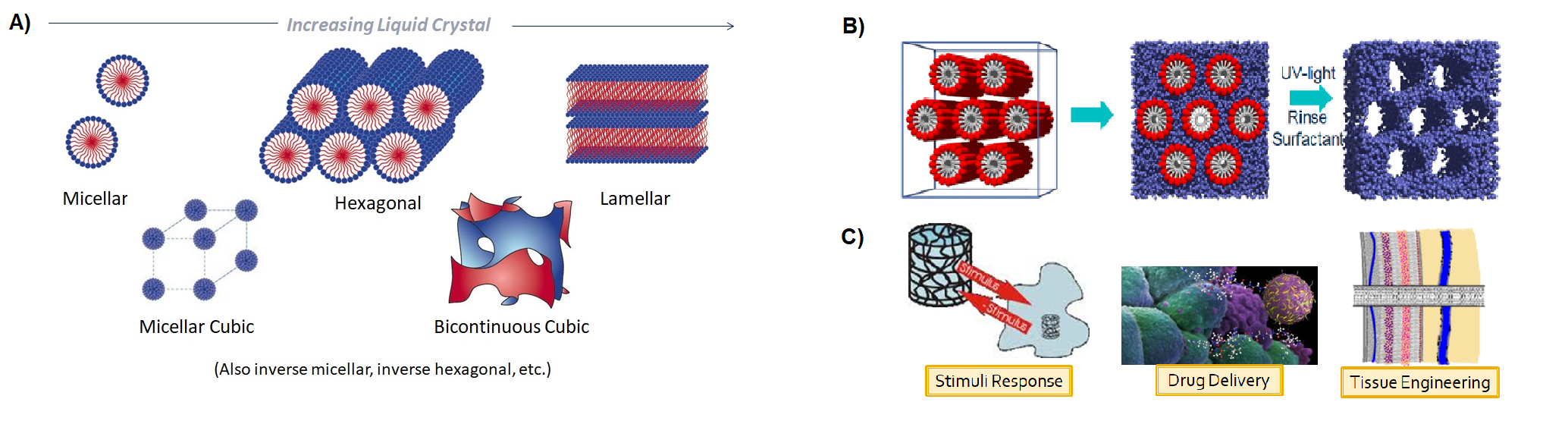
Improving 3D Photocured Systems
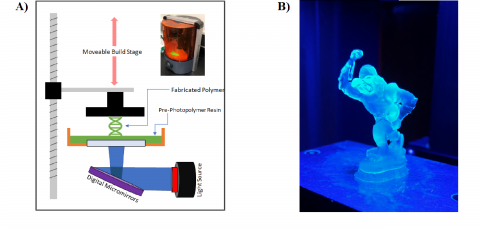
3D printing technology encompasses many additive manufacturing techniques for the fabrication three-dimensional structures. Among the methods of 3D printing available, stereolithography (SL) has proven particularly attractive.. With high resolution and the ability to rapidly produce prototypes with complex geometries, SL provides ease of product development that is unmatched by other manufacturing methods such as injection molding, extrusion, and subtractive processes. The specific method investigated by our group is digital light processing (DLP) SL in which a pre-photopolymer resin is selectively exposed to defined projections of near-UV light and cured layer by layer to form a three dimensional object (Figure 1). Although interest in this technology has significantly increased over the past few decades, widespread implementation of this technique is limited by the narrow range of mechanical properties possessed by polymers that it can be used to print. Specifically, SL-fabricated materials tend to be either brittle with low energy-absorption capabilities, or elastic with low structural integrity. In addition to these material constraints, the layer by layer fabrication results in anisotropic effects that can create weak points within the polymer matrix. Our group aims to address these issues through investigation of chemical and physical fundamentals governing the photopolymerization printing process.
Engineering Photografted Zwitterionic Hydrogel Coatings to Mitigate the Foreign Body Response to Biomaterials
The use of implantable biomedical devices such as pacemakers and artificial joints has increased dramatically in recent years, improving quality of life of countless patients. However, despite the advantages provided by biomedical implants, the formation of fibrotic tissue at the site of these implants poses significant challenges, particularly for neuroprosthetic devices such as cochlear implants (CIs). To address this issue, we investigate the use of zwitterionic coatings to reduce the foreign body response (FBR) to implanted biomedical devices. Zwitterionic polymers, which contain both positively and negatively charged groups, are hydrophillic materials that exhibit anti-adhesive/anti-fouling properties with respect to a range of biomolecules. Additionally, the high lubricity of zwitterionic coatings decreases the friction between biomaterials and biological environments. In this project, we seek to create coatings for CIs that will prevent the FBR and reduce fibrosis near the implant. Additionally, we anticipate that the results of this study may be extended to additional biomedical implants as well.
Directed Network Structure Through Controlled Radical Photopolymerization
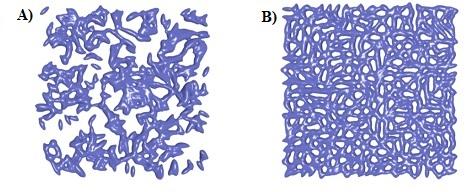
Acrylate monomers are popular in photopolymerization due to their fast polymerization rates and tunable structure. However, due to this rapid polymerization, acrylate-based materials are brittle and may have excessive shrinkage stress which may affect material toughness and durability. In traditional photopolymer applications, such as thin films or coatings, these shortcomings may be safely ignored or easily mitigated by modifying the monomer formulation or processing conditions. However, in thicker coatings the large amount of polymerized material may induce sufficient shrinkage stress to cause short or long-term material failure.
By adding chain transfer agents in photopolymer resins we are able to control polymer growth during photopolymerization to enhance homogeneity in photopolymers (Figure 1). I am currently focused on investigating the effects of chain transfer agents on photopolymerization kinetics of acrylate resins and characterizing final photocured materials thermomechanical properties.
Nano/MicroStructured Materials Obtained Using Photopolymerization-Induced Phase Separation (PhIPS)
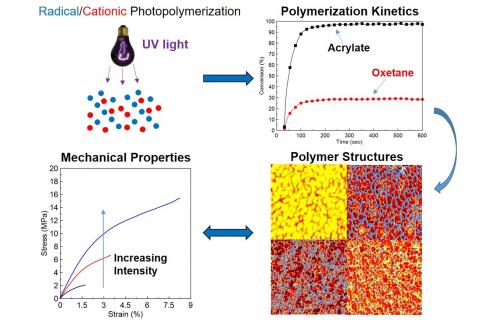
Controlled polymer phase separation enables significant improvement of a range of mechanical characteristics such as enhanced material toughness and impact strength, reduction of photopolymerization-induced shrinkage stress, and reduced material gloss. Photo-induced phase separation (PhIPS) is a method of photopolymerization in which phase separation is utilized to produce polymeric materials with tunable physical and mechanical properties. PhIPS can be controlled by altering photopolymerization conditions (e.g. light intensity) as well as formulation chemistry. As shown in Figure 1, differences in light intensity have been shown to significantly alter the formation of phase separated domains in acrylate-dioxetane systems due to differences in reaction rate of their constituent monomers. Differences in formulation composition also affect the interplay between phase separation and gelation onset in photopolymerizing systems as has been shown in studies examining the influence of compatabilizing hybrid monomers, crosslinkers, and oligomers in acrylate-oxetane resins.